
Here’s Why the Fusion Breakthrough—Decades in the Making—Matters
A tiny amount of energy but a very big deal.
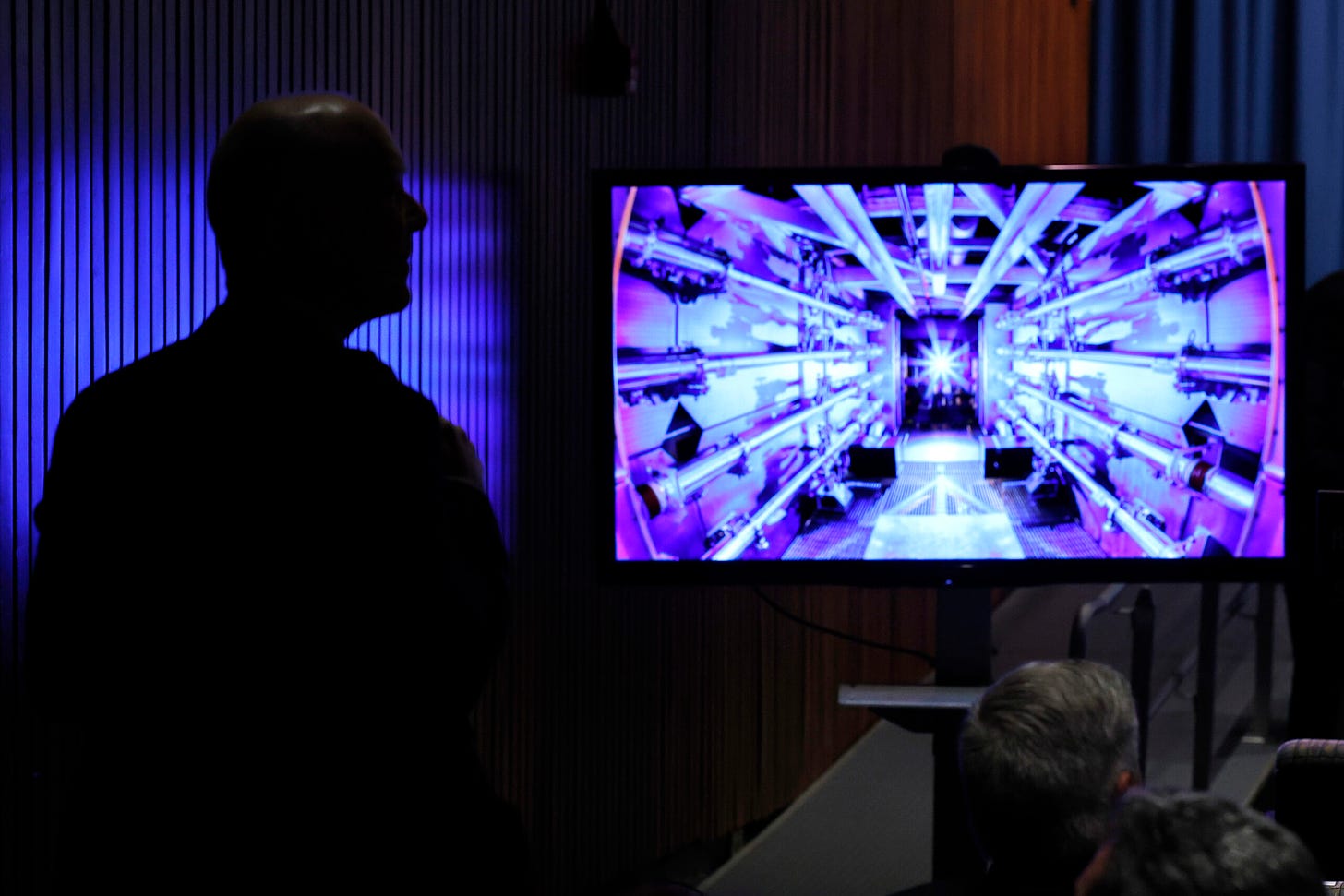
On Tuesday, Energy Secretary Jennifer Granholm announced that the National Ignition Facility (NIF), part of the Department of Energy–funded Lawrence Livermore National Laboratory, had achieved a milestone in fusion energy research. According to Granholm, on December 5, NIF had focused an array of 192 lasers into a cylinder holding a pea-sized pellet of deuterium-tritium fusion fuel and produced a thermonuclear reaction generating more energy than that used to drive it. The announcement was quick to set off headlines welcoming the advent of a vast new carbon-free energy resource for humankind, which were quickly followed by rebuttals from killjoys pointing out the vast distance between NIF’s accomplishment and a practical commercial fusion reactor.
Many of the points raised by the killjoys were correct. While the NIF fusion fuel produced 3 megajoules (a MJ is about the energy needed to power a 100-watt light bulb for 167 minutes) of energy after being hit by a 2 MJ laser pulse, it took over 200 MJ of electricity to drive the lasers. So, from a practical point of view, no net energy was produced. Furthermore, the NIF, a football-stadium-sized facility built at a cost $3.5 billion, does not remotely resemble a power plant. Despite its immense capital cost it includes no mechanism for translating its fusion output into electricity. Furthermore, even if its electricity consumption could be reduced to zero, at the yield demonstrated it would need to be fired a thousand times a second to generate energy at the rate of a major urban nuclear or fossil fuel electric power station. The best NIF can actually manage is about one shot per day.
Yet if the critics made some valid points, they missed the main event. NIF didn’t just achieve breakeven—getting more energy back that it put in—it achieved ignition. That is, they lit a thermonuclear fire in the lab. This has never been done.
To understand the significance of the NIF experiment, imagine that you are a Stone Age human, living in a society whose only source of fire is from lightning strikes. You observe that if you rub two sticks together they get warm. So you hit on the idea of rubbing them really fast and hard in order to try to light a fire artificially. After many tries and much effort, you manage to light a dry leaf on fire. The energy the burning leaf releases is much less than what you put in with your muscle power. But now you have a way to produce fire on demand. By analogy, that is what was just accomplished at NIF.
The road to the NIF breakthrough has been long and hard. Thermonuclear fusion reactions require temperatures far higher than that which any solid materials can withstand, so their ultra-hot fuel gas, known as plasma, needs to be confined without the use of solid containers. Stars can do it because they are surrounded by the vacuum of space, and can use their massive gravity to hold on to their fuel. Magnetic fusion devices, like the doughnut-shaped tokamaks, put tenuous plasmas in vacuum chambers and employ powerful magnetic fields to keep the plasmas from contacting the chamber walls. By contrast, inertial fusion devices, like NIF, attempt to avoid the necessity for either star-class gravity or massive magnets by heating the fusion fuel up so fast that it doesn’t have time to go anywhere before it can react.
The concept of generating a massive explosion using inertial fusion was first hit on by Enrico Fermi in 1941. The Manhattan Project ultimately focused on nuclear fission as a more practical way to create a weapon to win World War II. But Edward Teller became obsessed with the idea of using fusion to make a much more powerful explosive, and achieved success with the development of the hydrogen bomb in 1952. (The Soviets succeeded nine months later.) However, to obtain the power to heat and compress their fusion fuel fast enough to achieve ignition, H-bombs need to use fission bombs as triggers. Thus their minimum yield per detonation is that of their atom-bomb trigger, which is far too large to employ as a rapid-fire system for generating electric power. Furthermore, while the fusion reaction itself generates no radioactive waste, the fission bombs used as H-bomb triggers produce plenty of fallout.
If inertial fusion was to be used to produce power, much smaller explosions needed to be generated, and a way to trigger them without atom bombs had to be found. In 1960, John Nuckolls proposed triggering them using lasers, which were then still very new. Working at Livermore with Teller’s protégé Lowell Wood and others, by 1972 Nuckolls was able to publish a paper providing the math that showed it could really work. Serious experimentation attempting laser fusion soon followed.
In 1988, Nuckolls became director of Lawrence Livermore; during his five-year tenure, he advanced a proposal for a massive scale-up of laser fusion research by building NIF. While the DOE’s main drive for fusion reactors was focused on collaborating in the International Thermonuclear Experimental Reactor tokamak project, Nuckolls argued that using NIF thermonuclear-bomb physics could be researched without the need for actual underground bomb testing. This argument ultimately won the day with the Clinton administration and the NIF program was begun. Conservative calculations indicated that it would be best to design NIF to be able to deliver 10 MJ laser pulses to assure ignition. But there wasn’t the budget for that—2 MJ would have to do, making success chancy. The Lawrence Livermore team took the bet, and NIF was completed in 2009. For a decade and a half the team struggled to overcome all sorts of problems to make it work as they hoped. This year they finally pulled it off.
So now we have a way to produce fusion “fire” on demand. The knowledge we will gain from this capability will greatly benefit all other fusion efforts—including the inertial fusion program at the University of Rochester, which, limited by funding constraints to much smaller lasers than those employed by NIF, has developed a much more efficient approach to compressing and heating their fusion fuel targets.
A whole raft of entrepreneurial fusion efforts will likely be affected by this breakthrough as well. The success of SpaceX has demonstrated that is possible for well-led entrepreneurial efforts to enter fields that were once thought the province of superpower governments, and not only achieve their goals much faster and cheaper, but even develop technologies (such as rapidly reusable space launch) that were deemed impossible altogether. Taking their cue from this, heavy-hitting investors are betting that the fundamental problem with achieving fusion has not been so much technical, as institutional. Consequently billions of dollars of private money are now flowing into companies like Tokamak Energy, Commonwealth Fusion Systems, Helion Energy, and Tri Alpha Energy that are operating on development timelines measured in years, not decades. With the help of the data that will now flow out of NIF, they may reach that goal much sooner than many think.
Eighty years ago this month, on December 2, 1942, Enrico Fermi’s Pile-1 team achieved the first critical nuclear chain reaction under the University of Chicago’s original Stagg Field. Following this success, Arthur Compton, who was there representing FDR’s science advisory group, picked up the phone and called James Conant in Washington, saying, in an impromptu code, “The Italian navigator has landed in the New World.”
We’ve just landed in another.